Research

Research
We will research a number of beyond the state-of-the-art cooling system implementations and evaluate their performance under coolant conditions that would achieve acceptable life. We will research the innovative inclusion of transpiration cooling into jet engine turbine stage components and high endurance porous ceramics for hypersonic vehicles.
Within the spectrum of cooling systems considered, there are several permutations of cooling technology. Our work will enable us to rank such systems and inform their introduction by our colleagues in future jet engines and hypersonic vehicles. This over-arching aim will set the context of our work and keep the partners focussed on delivering real system benefits.
Develop Innovative Porous Materials

We will investigate hybrid methods of manufacturing superalloy turbine blades and develop varying porosity ceramic materials. For turbine blades, our starting point will be manufacturing fabricated double skin systems. This work will utilise the new HTRC facility to deliver rapid high quality castings and process innovation. We will research Ultra High Temperature Ceramics (UHTCs) to include controlled porosity to be used with transpiration cooling for specific application to hypersonic vehicles. These UHTCs are capable of operating at temperatures over 2200 K, well in excess of carbon composites investigated to date for hypersonic transpiration cooling, and will build upon work from the XMAT programme. For both solution, the challenge will be to maintain the excellent thermal and structural properties whilst moving to high porosity.
Experimental Verification

We will perform experiments to study the success of selected transpiration systems. This research will take advantage of transient experimental techniques developed at University of Oxford to measure heat transfer, metal effectiveness and film cooling effectiveness while matching flight or engine non-dimensional flow properties and geometries. For turbine blades, the research will combine metal parts with scaled take-off conditions achieving simulation of film to gas density ratio using foreign gas or engine gas to coolant temperature ratio. For hypersonic vehicles, we will couple the high speed conditions achievable in the short duration facilities at University of Oxford (part of the National Wind Tunnel Facility, NWTF), with directly heated transpiration cooled porous models. In parallel, testing will be performed at the long duration facilities at Stuttgart University to achieve thermal equilibrium at correct heat fluxes - without simulating the flow field.
Numerical Method Development

Our research will combine experiments in the high speed facilities at the Oxford Thermofluids Institute whilst simultaneously advancing CFD methods to provide a full understanding of transpiration cooling and design capability. A multi-scale approach will be used to develop numerical methods to model and understand the basis and the implementation of transpiration cooling. This research will build on the Fourier spectral approach and the Immersed Mesh Block technique developed at the University of Oxford and extend these to conjugate CFD predictions for intricate cooling features. The accuracy of these calculations will be assessed against both fundamental and applied experiments using measured specific thermal properties.
Lifing Model Development
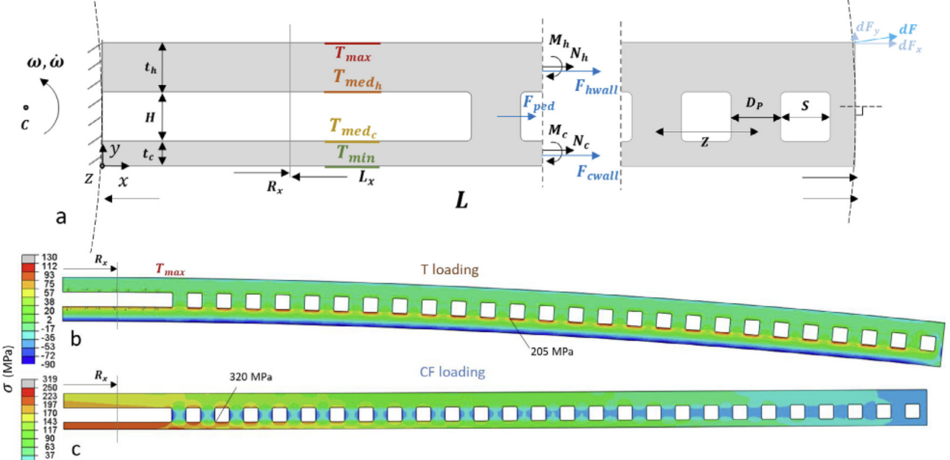
We will develop an in-depth understanding of potential failure mechanisms in the different types of transpiration system covered by this grant and evaluate the degree to which direct and indirect computational procedures can be employed to facilitate their design and optimisation. By combining analytical and numerical models with selected tests, we aim to determine the key mechanical and physical material properties required for materials to be used in future transpiration systems.
Transpiration Flow Mixing
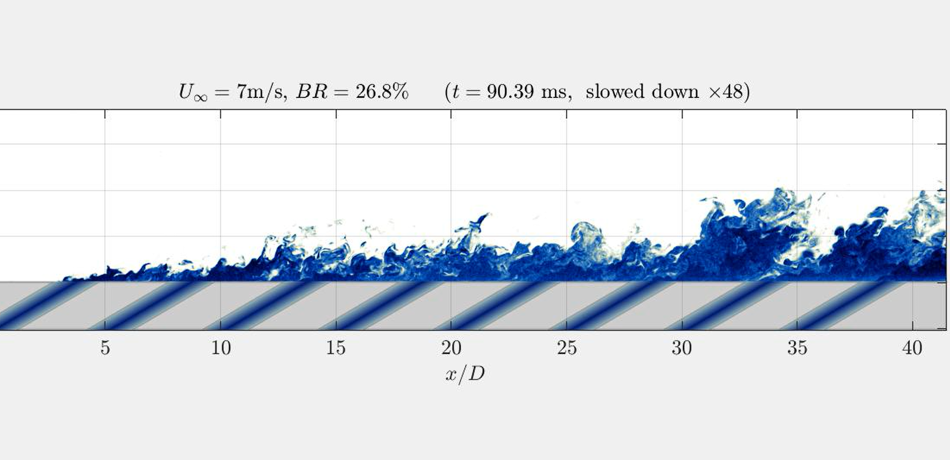
We aim to develop a complete understanding of the flow parameters that determine flow mixing for films in transpiration cooling. This research will use large scale experiments in a NWTF wind tunnel at Imperial College and advanced laser diagnostics to provide information into cooling film development in the turbulent boundary layer at unprecedented spatial resolution. The idealised experiments will match the important dimensionless groups and provide fundamental understanding and validation data for turbulence and flow mixing modelling.